Photonics Research, 2020, 8 (7): 07001213, Published Online: Jun. 30, 2020
Multitask deep-learning-based design of chiral plasmonic metamaterials
Download: 621次
Figures & Tables
Fig. 1. Schematic of (a) a single YNP chiral meta-absorber array with definition of incident circularly polarized lights and a unit cell with dimensions. (b) Three single YNP metastructure configurations: Au YNP/Glass (YG), Au YNP/PMMA/Glass (YPG), and Au YNP/PMMA/Au/Glass (YPAG). (c) Absorption and CD spectra of the three metastructure configurations (YG, YPG, and YPAG), showing their plasmonic resonances λ p (λ p = 620 nm , 625 nm, and 645 nm, respectively) and revealing a strong chiroptical response for the metamaterial absorber case (YPAG). Here, R 0 = 100 nm , t 1 = 40 nm , t 2 = 50 nm , t 3 = 100 nm , and t 4 = 200 nm .
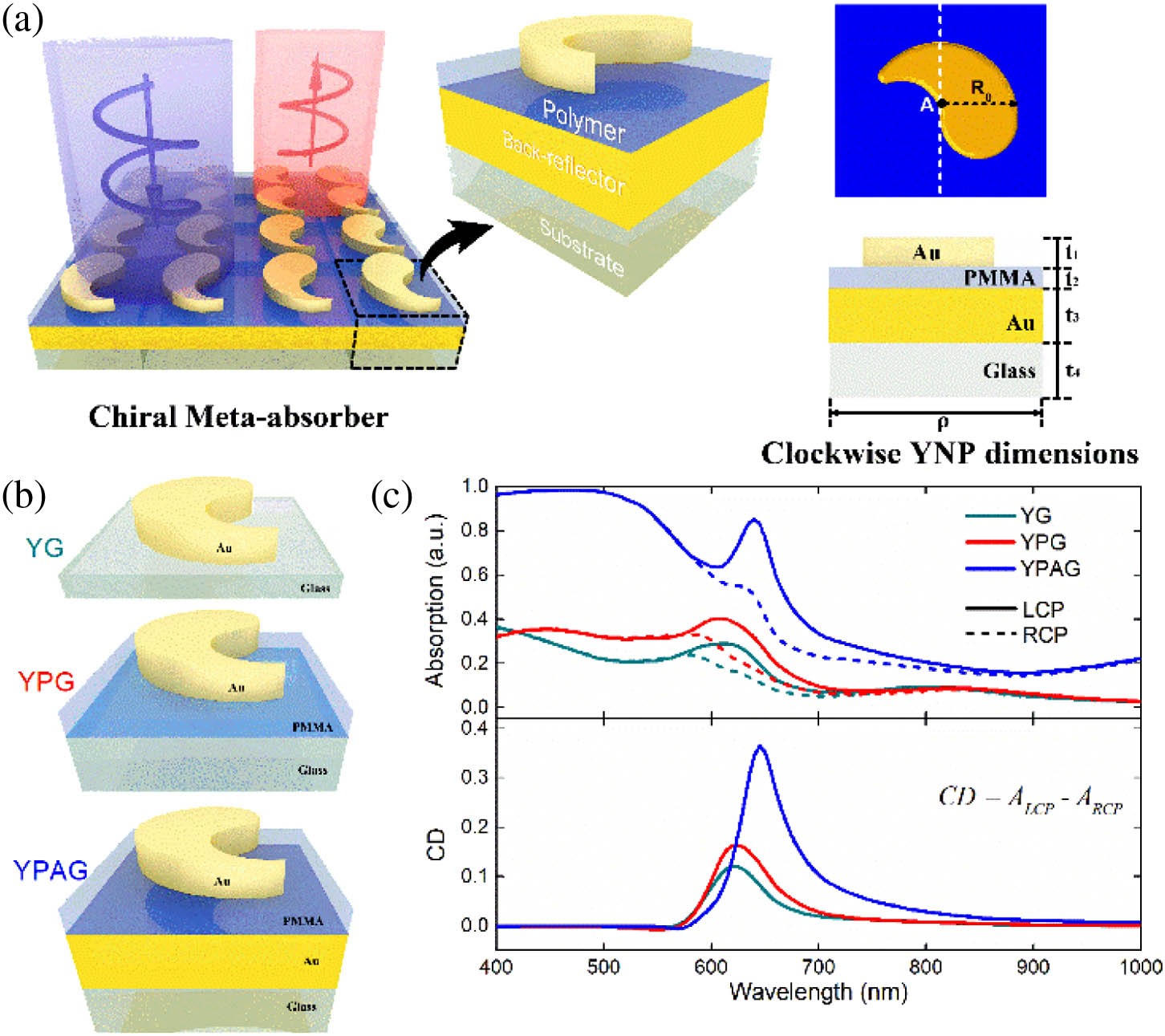
Fig. 2. Schematic of the bidirectional multitask deep-learning model for chiral metamaterial design consisting of forward design path (FDP) and inverse design path (IDP). Each path is composed by shared layers and task specific layers with joint optimization functionality. The model is set up in an end-to-end fashion where the geometric design parameters, CD, and LCP/RCP absorption spectra can be treated as input or output at specific ports. Here, the geometric design parameters are the YNPs thickness, PMMA thickness, YNPs radius, and YNPs (respectively represented as x i , i = 1 , 2 , … , 6 ). x 4 has been taken as a constant in the data shown in this study, but is nonetheless included in the model to represent the general parametrization of the system. The inset shows the metamaterial absorber geometry used to exemplify the use of the MDL.

Fig. 3. MDL model performance. (a) Numerical simulation and (b) MDL prediction CD results of the dimer structure at varying gap distance d (50–160 nm), across the visible and near-IR regime. R 0 = 100 nm , t 1 = 40 nm , t 2 = 50 nm , and t 3 = 100 nm . (c) Numerical simulation and (d) MDL prediction results of the dimer at varying YNP thickness (t 1 ). The color legend has been truncated at ± 0.2 for clarity. Inset, definition of the gap d . (e) Learning curve within 3000 epochs. (f) Discretized model performance at selected t 1 = {60 nm, 80 nm, 100 nm} corresponding to the horizontal dots in (c) and (d). (g) Model performance at λ 0 = 755 nm across varying t 1 , corresponding to the vertical short dashes through (c) and (d). Here, R 0 = 100 nm , d = 100 nm , t 2 = 50 nm , and t 3 = 100 nm .

Fig. 4. MDL-predicted CD progressions. (a) CD evolution by varying YNP radius at t 3 = {100 nm, 150 nm, 200 nm}. CD map plot by varying concurrently, (b) YNP radius and polymer thickness at t 1 = {5 nm, 25 nm, 50 nm} for λ 0 = 780 nm , (c) YNP thickness and polymer thickness at R 0 = {100 nm, 150 nm, 200 nm} and λ 0 = 700 nm , and (d) YNP radius and YNP thickness at t 2 = {10 nm, 45 nm, 100 nm} and λ 0 = 650 nm . The color legend has been truncated at ± 0.2 for clarity, but high-CD areas have been highlighted by adding the contour regions corresponding to CD values of 0.5.

Fig. 5. Inverse design with the MDL model. (a), (b) Simulated (green solid lines) and predicted (red dots) CD spectra. (c), (d) Corresponding simulated (green bars) and retrieved (red bars) geometric parameters. Red dots in (a), (b) are predicted from the MDL model with geometric parameters retrieved [red bar in (c), (d)] for the target simulated CD spectra in (a), (b). [See Figs. 9(a) and 9(b) in Appendix C for absorption spectra comparison.]

Fig. 6. Enantiomer detection. (a) The CD spectra of (red) left-handed medium (LH m ), and (blue) right-handed medium (RH m ) with molecular CD resonance (λ m = 380 nm ) in the UV. (b) CD spectra comparison of the right-handed chiral metamaterial absorber (RH cma ) with (blue) and without (red) chiral medium (CM). Inset is the electric field at the plasmonic resonance, λ p (λ p = 665 nm ), of the bare chiral metamaterial absorber for LCP and RCP light. (c) CD summation to remove metamaterial background CD signal to reveal the LH (blue solid line) and RH (green solid line) enantiomer pair CD signals. λ m , λ p , and λ m p represent the resonant wavelengths for the CD of the bare molecules, the plasmonic chiral metamaterial absorber, and the metamaterial covered with chiral media, respectively. Inset, schematic representation of an enantiomeric protein molecular pair (L and D isomers). (d) Electric field, surface charge density, and optical chirality density distributions of the chiral metamaterial absorber covered by chiral media (CM) at λ m p = 720 nm .

Fig. 7. Electric field and surface charge density distributions of the three single YNP metastructure configurations (YG, YPG, YPAG) at their plasmonic resonances, showing huge enhancement for the metamaterial absorber case. The cut plane is through point A, the unit cell center [Fig. 1(a) ].

Fig. 8. Optical and chiroptical properties of the chiral metamaterial absorber with 10 nm tip rounding radius. (a) Absorption and (b) reflection spectra of single yin-yang metamaterial absorber at varying polymer thicknesses. Corresponding (c) CD and (d) g CD from (a) exhibiting a strong peak in the visible regime (around 635 nm wavelength). CD response from three gapped configurations of the chiral metamaterial absorber: (e) dimer, varying gap length d , (f) circular, varying PMMA thickness, and (g) circular with central disk, varying central disk radius r . (h) Charge density distribution at the CD maxima (peak A) for the 60 nm PMMA thickness in (c).

Fig. 9. Inverse design plots. Simulated and MDL-retrieved absorption spectra comparison for the systems providing the data in (a) Fig. 5(a) and (b) Fig. 5(b) . (c) Comparison between the CD values obtained with different variations of the ML techniques: separately training LCP and RCP spectra (black dashes), training only CD without auxiliary tasks (red dashes), training by our MDL model (blue dashes), and the ground-truth (pink solid line). The inset is a zoomed-in image at the resonances.

Fig. 10. MDL-predicted CD progressions. CD evolution when varying PMMA thickness at t 1 = {30 nm, 60 nm, 90 nm} for R 0 = 100 nm . This plot agrees with the Fig. 3 , where we observe only CD maxima for t 1 = 30 nm but both CD maxima and minima for t 1 = 60 nm and t 1 = 90 nm , establishing the consistency of the MDL model.

Eric Ashalley, Kingsley Acheampong, Lucas V. Besteiro, Peng Yu, Arup Neogi, Alexander O. Govorov, Zhiming M. Wang. Multitask deep-learning-based design of chiral plasmonic metamaterials[J]. Photonics Research, 2020, 8(7): 07001213.